As a design engineer, when I have pivoting members, one of my favorite bearings to use are fiber reinforced bearings. They are easy to apply and are very predictable.

Image courtesy of GGB
Fiber reinforced cylindrical bearings are round bearings with no moving parts. The inside diameter is coated with a low friction surface that is usually either nylon or Teflon. Carbon fiber is then wound on the outside to give ample strength.
To properly apply a cylinderical bearing an engineer must address all of these:
- Selecting the size and wall thickness
- Calculating the bearing pressure
- Determine frictional forces
- Calculate surface velocity
- Estimate life cycle
- Identify and minimize misalignment
- Assembly Considerations
- Common Issues
Let’s dive deeper:
Fiber reinforced cylindrical bearings are a subset of cylindrical bearings, but as mentioned, they have no moving parts. Typical cylindrical bearings have an inner and outer race, balls or rollers and seals. Having no moving parts makes the design more robust.
Are they useful in engineering design and if so, how useful?
As I look back on the last 15-years of my design career, cylindrical bearings stand out as being one of the most reliable and commonly used items in nearly all of my projects. They can be trusted to perform.
Technically, they are classified either as filament wound, self-lubricating bearings or simply by their brand names such as GarMax or Polygon. These bearings are unique because they have no moving parts.
The outside diameter is pressed into a bore and the pin is able to rotate on the inside diameter of the bearing. The bearings are formed by first putting a wear material like PFTE (Teflon) on the surface and then a winding material like carbon fiber or fiberglass on top. These bearings are a good choice for their:
- High load capacity
- Inexpensive pricing
- High temperature capability
- Minimal to no maintenance
- Long life
- Small form factor
The main reason to steer away from these is high surface velocity.
Like any other bearing, there are several important things to keep in mind in the design:
1. Selecting the Size and Wall Thickness
Cylindrical bearings are specified in 16th of an inch size and come in 1/8 and ¼” wall sizes (metric versions are also available). For example, if we were specifying a GarMax bearing of GM1620-16 the form is the:
- Product code -GM
- Inside Diameter, ID – 16 (1 inch)
- Outside Diameter, OD – 20 (1.25 inch and thus a 1/8 wall)
- Width – 16 (1 inch)
This is great because if you want to change to another type or supplier, you just change the product code using the formula above. It’s simple enough that you could extrapolate that to get other sizes.
Cylindrical Bearing Thickness
There are no hard guidelines here. I have found that if the bore size is fixed, lean toward a 1/8” wall bearing. This allows you to use a larger pin with more strength.
I learned this from a custom cylinder application were the loads were high and caused damage to the pin. Because of part interchangeability, we were not inclined to change the rod eye size of the cylinder, but we found that if we changed the bearing from a ¼” wall to a 1/8” wall we could use a ¼” larger pin.
This brought our design factors back up to allowable limits.
If the bore size is fixed, lean toward a 1/8” wall bearing. If the pin diameter is fixed I recommend a 1/4” wall bearing.
If the pin diameter is fixed and the bearings are pressed in a tube, I would recommend a 1/4” bearing.
Tubing is readily available in 1/8” steps of wall thickness and in DOM tubing (the good stuff) up to 5/8” thick. This allows me to machine my bore about 1/4” bigger than the ID on only a little bit deeper than the bearing depth on each side.
The thicker bearing prevents the need for cleaning up the tube’s stock ID between the bearings. As a result, I can machine this bore up to 1/8” off center (eccentric) and still not have any interference with the pin. This can be a big time saver in your machine shop.

2. Calculating the Bearing Pressure
Bearing Pressure (P) — Calculating the pressure for the bearing is important. First, find the area by taking the inside diameter and multiply it by the width. This number is a representative stress because the edges see no load and the center sees most of it. Let’s make our area one square inch.
We calculate the pressure by taking a force (1000 pounds), and divide it by the area (1 square inch) so our answer is 1000 PSI.
The bearing manufacturers have guidelines for the allowable pressures for many different applications. Generally they range 5000 psi 10,000 psi and some can be higher.

3. Determine the Frictional Forces
Calculating the friction for the joint is also important. We will need to determine the frictional properties of the design and determine how much torque it is going to take; under load, to rotate the pin. And that’s critical if you’re going to size a cylinder or hydraulic motor that forces the motion. The general formula for calculating torque is equal to

T is torque in in-lbs., F is the force in lbs., and D is the diameter of the pin in inches. The diameter is divided by 2 for this reason: You are going to be rubbing on the bottom edge leaving a slight gap at the top. As a result, the pin will press at the center of the bearing at half the diameter.
The chart below is typical of cylindrical bearings. You can see from the chart below that as you increase pressure on the bearing, your coefficient of friction actually goes down.

This is a good thing because it means that as I load the bearing up more and more it actually gets easier to turn. But be cautions. If the bearing is oversized, pressures will be low (below 5000 psi), I can actually have a bearing and that is very hard to turn or worse it makes a lot of noise because low pressure leads to unwanted vibration creating unwanted noise.
I was once party to a design that had a bearing that was so loud it sounded like an irate whale. And you could hear it from half-a-mile away. We ran the calculations on it and found out that the pressures were around 1800 psi (very low). We narrowed up the bearing to increase the pressure and the noise was gone! So when you’re sizing bearings make sure that you look at the highest load and lowest load case.
4. Calculate Surface Velocity
This is the speed at which the pin surface sees movement. This is usually measured in feet per minute (fpm). So to convert from revolutions per minute (rpm), use the equation below. The 12 is a conversion from inches to feet so be sure to check your units.

Where V is the velocity in fpm, n is the rotation speed in rpm, and D is the diameter in inches. These types of bearings generally have a cut off speeds between 10 fpm and 25 fpm.
PV – The next consideration is a PV factor. It is simply the product of pressure and velocity. It is essentially an empirical method of estimating the bearing life. It balances the force and pressure to a limit. For example if our limit is 36, we could have a pressure of 4 and a velocity of 9 or a velocity of 4 and a pressure of 9. So we now have three limits on our bearing.
5. Estimating Life Cycle
Once we have the PV value, we then can calculate the estimated life cycle. What is life cycle? The life cycle is a function of multiple factors. Most of them can be looked up in the manufacturer’s charts.
- Cyclic life factor (Q) — This is provided by the manufacturer based on the bearing type
- High load factor (aE) — This is a derating factor if the pressure is on the high end of the spectrum
- Temperature factor (aT) — Heat dissipation is important. If the ambient temperature is elevated, a derating factor is needed.
- Pin surface factor (aM) –The manufacturer will give you a table to look up this factor. Generally a surface finish between 6 and 16 micro-inches is preferred.
- Pin surface finish factor (aS) — Even a little roughness on the pin surface can act like sand paper and de-rate the bearing life.
- Bearing size factor (aB) –This factor looks at the angular pressure area. This factor decreases as the bearing diameter increases because the used area of the bearing gets smaller and smaller as diameter increases.
As mentioned, there are charts and tables where all this information can be found. To calculate the lifecycle (in cycles) you combine all those elements as shown below.

6. Identify and Minimize Misalignment

It’s a bearing’s worst nightmare when things don’t line up! This can be remedied by proper machining, mechanical adjustments, or welding with the pin already in place.
Misalignment is measured in percent. A 0.2% misalignment is defined as 0.002” per inch of bearing. This level of misalignment is standard and no adjustments need to be made to the bearing pressure. A 0.6% misalignment is the highest I would ever consider allowing.
No matter what your P value is, your edge load stays constant. For example, if I have a bearing with diameter 2”, width 2” and loaded to 10 ksi, I will have an edge loading of 34 ksi. Quite a multiplier!
If you have a bearing that supports a cantilevered load, you are almost automatically at a 1% misalignment. That is a lot! You are essentially expecting 0.010” of deflection in the joint.
The edge loadings can easily double or triple the calculated P value. In the example above, a 1% misalignment would yield an edge loading of 45ksi. Ouch!
I highly recommend changing a cantilevered design to include two bearings that are spaced apart so that the bearing takes two axial loads rather than a moment load. You will also reduce the friction that will resist motion in the joint.
7. Assembly Considerations
- Bore sizes – Over the years I’ve had a hard time reconciling the fact that the bearing can come in with an outside diameter tolerance of 0.002 to 0.004, but I have to hold my bore to 1/3 or ¼ of that. It’s not fair! Honestly, I’m not sure how it works mathematically. But it does! I’ve used thousands of these over the years in dozens of different applications and when machined to the recommended bore sizes you will not have any problems with assembly or undue wear. Opening up tolerances is usually a good cost savings so beware of attempts to change this.
- Pin sizes – The same is true with the tolerances on pins. We get a little help on this one, though. If you have read my article on pins, you will see that I recommend the use of turned, ground and polished pre-chrome rod. The manufacturers know that the TG&P rod comprises a large portion of the pin industry and as a result, the TG&P tolerance matches the manufacturer’s recommendation (Wow!)
- Chamfers – When installing a bearing, I recommend adding chamfers to both the pin and the bore. I recommend a 15°-30° x 1/16 (on the diameter) chamfer to ease alignment.
- Bearing knockers – If you are assembling this bearing in high quantities, I recommend designing a bearing knocker. The knocker is simply a steel component that matched the ID of the bearing. It has at least one step to provide good contact on the edge of the bearing when pressure is applied. The matched ID prevents the bearing from having any localized buckling. A second step may be added if the bearing is to be recessed past the surface of the bore. You may need this to get past your leading chamfer on the bore.
8. Common Issues with Fiber Reinforced Cylinderical Bearings
As will all things, there are several things that are bound to happen when applying fiber reinforced bearings. Here are the most common.
- No lead in chamfer – Add a lead in chamfer of at least 1/16” deep. I recommend a 15° angle so that the bearing has a smooth guide. You must also insert the bearing at least as far as the chamfer to make sure the bearing is properly supported.
- Not cleaning out the bore – Frequently, paint overspray or other debris can mess with bore. It doesn’t take much to make a negative impact to the design. Use a flapper wheel tool to remove the paint, but don’t go crazy. You machined that hole for a reason!
- Improper bearing installation – As mentioned, a slight press fit is recommended between the bore and the outside diameter of the bearing so it is going to take some force to install. The most common method is using a hammer. This one makes me cringe a bit. There are so many things that can go wrong here.
- Even pressure distribution – There is no real way to make sure that a hammer blow is going to contact the edge evenly. This can partially be avoided by having the hammer strike a flat piece of wood larger than the bearing.
- Buckling – This is a thin walled tube we are dealing with and it will buckle. Unlike steel, these bearings are anisotropic so when the compression loads are too high, the fibers will separate. At this point, the bearing must be replaced. As with all buckling, the best solution is to add more support over the length. I recommend having a bearing knocker (blue part below) that will add support all along the inside of the bearing. This nearly eliminates all buckling issues
- There is an unknown amount of force that the hammer can produce. For large bearings, this can be properly sized, but for small bearings, you are likely to damage the bearing. I have one recommendation for this; use a bearing press. If you can’t get the piece to a bearing press, you can make one with a hollow cylinder (enerpac.com) and some all thread. Simply insert the all thread through the bore, bearing, knocker, cylinder and a few (thick) plates with holes in them. Add some nuts on the all thread until snug. Then use the cylinder to push the bearing into the bore.

Final Thoughts
Calculating cylindrical bearings can be a little tricky. To speed up the learning process, I have an example problem worked out for you.
I’ve also developed an online calculator for a quick check to see if your calculations are correct. It calculates pressure, velocity, torque and estimated life (including the factors). You will need to be able to verify and interpret the results for the brand of bearing you’re using but it will save you time by calculating most of the information.
In conclusion, cylindrical bearings are wonderful. They allow high loadings in tight spaces. They are largely chemical resistant, do not require lubrication and do not break the bank. When designing, seek these bearing products out! Remember, almost everything I design uses at least one of these bearings.
Enjoy designing!
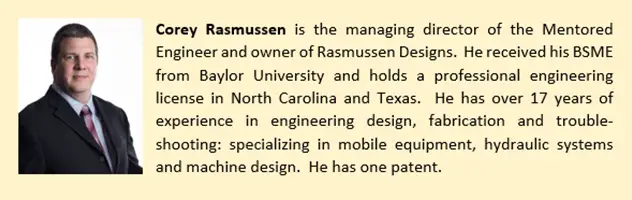